|
 |
Enter subhead content here
|
 |
The Ford Flathead Engine 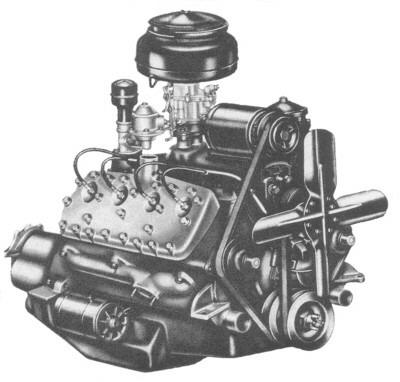
How Too Size A Turbo-Charger
For Our Application...
Project: Flathead is going to be Turbo-Charged, so we need to know how to choose the correct Turbo for the engine we have.
Were starting out with a Ford Flat-Head Engine, it has 235 ci, 8-Cylinders and is a 4-Stroke. Configuration = V-8 Cubic Displacement = 235 ci Bore = 3.1875" Stroke
= 3.750" Ambient Temperature = 70°f Barometer = 29.0 in Hg Maximum RPM (a) = 5,000 rpm
(b) = 6,000 rpm Maximum Boost Pressure
(a) = 7psi (b) = 14.2 psi I will be tracking the conditions of two separate scenario's, I think this will add
Balance and allow some interesting comparisons. Let's start by Converting the Displacement of our Engine from cubic inches to cubic feet Converting the Displacement
235ci
= 0.1359 cu ft
1728 cu in/cu ft Airflow Rate - Airflow
rate through an engine is referred to as cubic feet per minute (CFM) at a standard atmospheric pressure. Next we will Calculate the
Ideal Volume Flow through our Engine Ideal Volume Flow Engine
(a) 0.1359 cu ft x
5000 rpm = 339.75 cfm Revolutions 2 Engine (b) 0.1359 cu ft x 6000 rpm = 407 cfm Revolutions
2
These figures above are at 100% Efficiency, because of Residual Gases, Intake and Cylinder Head restrictions most
Engines don't operate at 100% Efficiency, a more realistic figure for our Engine would be 80% Efficiency. Converting to 80% Efficiency Engine (a) 339.75 x .80
= 271.8 cfm Engine
(b) 407 x .80 = 325.6 cfm Another way to determine the Air Flow rate is by the following: Airflow rate = CID x rpm x 0.5 x Ev (80%)
1728 Engine (a) Air Flow
Rate = 235cid x 5000rpm x 0.5 x 0.80
1728 Air Flow Rate = 470000
= 271.99 cfm
1728
Engine (b) Air Flow Rate = 235cid x 6000rpm
x 0.5 x 0.80
1728 Air Flow Rate = 564000
= 326.38 cfm
1728 With this method, the flow rate is in cfm and the displacement
is in cubic inches. We use .5 because the 4-stroke engine only fills the cylinders once every two revolution,
and the 1728 converts the cubic inches to cubic feet. Both methods will work and both give the Air Flow Rates of Normal,
Turbo-Charged or Super-Charged engines, based on how much air an engine will flow at 80% efficiency. All compressors are rated at Inlet Flow, and air entering
the intake will not be at standard conditions (29.92 in Hg & 60°f) Because of this, it is necessary to compute
the actual density to determine the air flow through the turbochargers compressor. For our application, we will consider
compressor Efficiency to be 65%. First we need to find our Pressure Ratio. Pressure Ratio Pressure Ratio = Manifold Pressure Absolute Inlet
Pressure Absolute (To convert from psi to in Hg you need to
multiply by 2.03) Engine
(a) PR = (7psi
x 2.03) + 29.0 = 1.49
29.0 Engine (b) PR = (14.2psi x 2.03) + 29.0 = 1.994
29.0 We got our (Y) figures from the Ideal Temperature
Rise Chart Engine (a)
Y = 0.121 Engine (b)
Y = 0.217 It is also necessary
to add 460f to the temperatures because the calculations need to be in Absolute Temperature (degrees Rankine) Tideal = Y x T1
Engine (a) Tideal = 0.121 x (70 + 460) =
64.13f Engine (b) Tideal
= 0.217 x (70 + 460) = 115.01f Actual Temperature Rise Tactual = Tideal / Compressor
Efficiency (65%) Engine (a) = 64.13f / .65 =98.66f Engine (b) = 115.01f / .65
=176.93f Intake Manifold Temperature IMT
= Compressor Inlet Temperature + Tactual Engine (a) = 70°f +
98.66°f = 168.66°f Engine (b) = 70°f + 176.93°f = 246.93°f Notice
the huge difference in Temperature between the two (2) engines? Remember, the engines are identical, except for the
higher boost and RPM levels. Engine (b) would absolutely benefit from some sort of Inter-Cooler. These Intake Air Temperatures may or may not actually get this hot. There
is some cooling effect from the Inter-Cooler tubing, and because fuel itself has an cooling effect on the intake air.
Project:Flat-Head will include the use of Twin-Inter-Coolers,
which will substantially lower this intake Air Temperature. But we have to figure the Theoretical Intake Air Temperature
to get the Density Ratio. Density
Ratio Turbo-Chargers
produce there power by packing more air molecules into the combustion chamber. How tight these molecules are packed
into each cubic inch of volume, is refereed to as the Density of the Air Charge. The Density Ratio is nothing more then comparing the Inlet Temperature to the Outlet temperature,
and the Inlet Pressure to the Outlet Pressure. Density Ratio
= Inlet Temperature
x Outlet Pressure Outlet
Temperature Inlet Pressure Engine
(a) Density
Ratio = 70 + 460 x 14.21+ 29.0
168.66 + 460 29.0 Density
Ratio = 530 x 43.21
628.66 29.0 Density Ratio =
0.84306 x 1.49 Density Ratio = 1.256 Engine
(b) Density
Ratio = 70 + 460 x 28.826 + 29.0
246.93 + 460 29.0 Density Ratio =
530 x 57.826 706.93
29.0 Density
Ratio = 0.7497 x 1.994 Density Ratio
= 1.494 Compressor Inlet Flow Now with this piece of the puzzle solved we can calculate the Actual Compressor Inlet Flow,
under these conditions. Compressor Inlet Flow = Compressor Outlet Flow x Density Ratio Engine (a)
Compressor Inlet Flow
= 271.8 cfm x 1.256
= 341.38cfm Engine (b)
Compressor
Inlet Flow = 326 cfm x 1.494
= 487.04cfm
|
 |
We now have everything we need to find the Turbo-Charger that is right for our engine. Most
legitimate Turbo-Charger retailers will display the Compressor Map for each Turbo-Charger they sell, same goes for the manufacturer,
they should make available each Compressor Map for the various Turbo-Chargers they sell. With the data now "in
hand", you can compare the many different Turbo-Chargers so you find one that works best for your situation. Also,
when doing calculations like these that take into account the Ideal Temperature Rise, where you need the "Y"
Data from a table, many times this table and it's data will be located on the manufacturers or retailers site. If
not, call them and they can provide it for you. Most
Compressor Maps are in cfm, some are in lb/min. Too convert cfm to lb/min simply multiply the cfm figure
by 0.069. Also note that most Compressor Map created for lb/min, are also calculated with the Turbo-Charger at 85°f
and a barometer of 28.4 in-Hg. Lets look
back and see what we have found through all these calculations.
On our Project Engine (a)
Approximately 49% more air will be going through the engine
then the engine could have consumed by itself. Engine (b)
will have approximately 99% more air. We find this out
my looking at the Pressure Ratio. Pressure is also measured in bar, and Atmospheres (ATM) ,1 ATM = 1 bar = 14.7psi.
In engine (b) the the PR or Pressure Ratio, of 1.99 is equal
to 1.99 bar. In theory, engine (b) would have an equal
power output of an engine twice the displacement !! that means our little 235 under 14.2 psi of boost, would act like a big
block 470 engine at atmospheric. Kind of like dynamite, a big bang in a small package. Project:Flat-head
is going to be a Twin Turbo-Charged engine, so we need to calculate a few more items. It a huge misconception
to think Twin turbo-chargers are inherently more powerful then a single turbo set-up, as too many other factors are involved.
On in-line 4-6 and 8 cylinder engines a single turbo may in-fact be the best way to go. Usually over 350cid, and on
any "V" type engine design, the dual turbo set-up is necessary. The particular arrangement of the V-8 0r V-6
engine makes twin-Turbo's more of an attractive lay-out in terms of not only aesthetics but also thermal efficiency.
A lot of energy (heat) is lost trying to tie the two exhaust manifolds together for a single turbo lay-out. Space and
Plumbing need also be considered.
Turbos produce Huge amounts of Torque - and Torque is FUN!!
On Project:Flat-Head we are using Twin T3/T4 T04E Turbo-Chargers. These HYBRID turbochargers consisting of a T3 turbine section, (standard, Stage
II or Stage III trim) and a T4 compressor section (T04B trim or T04E trim). The T3/T4 HYBRID’s offers the low inertia
and fast boost response of the light weight T3 turbine wheel and the high airflow characteristics of the T4 compressor family,
making the T3/T4 HYBRID the turbo of choice for high performance applications. Thats all great, but what is LOW INERTIA
and FAST BOOST RESPONSE ?
Inertia
- The tendency of a body to resist acceleration; the tendency of a body at rest to remain at rest or of a body in straight
line motion to stay in motion in a straight line unless acted on by an outside force. Resistance or disinclination to
motion, action, or change.
On a turbo engine we have to consider the time it takes for the compressor to get going fast enough to make boost. This
small delay between the throttle opening and the engine making boost is know as Lag, and inertia has everything to do with
it. As the definition describes inertia, it's the resistance to change. And when you open the throttle, you
want change, and quickly! Lets look at a few different scenarios. Moment of inertia is the resistance of a rotating body to a change in speed, represented by the letter
I. I = K2M where K is the radius of the gyration and M is the mass of the body. Radius of gyration is the distance from
the rotating axis to the point where all the mass of the body could be located to have the same I as the body itself.
In other words,
a 12-inch diameter turbine wheel might be represented by a ring with a diameter of 7 inches. In this case, K = 3.5 inches.
For good rotor acceleration it is important to have the smallest moment of inertia possible. Most turbine wheels are
designed with minimal material near the outside diameter to reduce K. The moment is proportional to the square of K,
so reducing K by 1/2 will reduce 1 to 1/4 of the previous value. Because of this, it is often to your advantage to use two (2) smaller turbos rather then one (1) larger turbo. Example: On Project:Flat-Head we are using two (2)
turbos and the major diameter of one of the turbine wheels is 2.319" with an assumed weight of .89 lb's. the
computation works out as follows. I =
K2M I = K2wg
1.15952 x .89lb
386 I = 0.003099 in-lb-sec2 If
we were to use a larger single turbo set-up the out come would look more like this, a single T-72 with a 4.030" turbine
wheel and a weight of 1.65lb. I
= K2M I = K2wg
2.0152 x 1.65lb
386 I = 0.017355 in-lb-sec2 An increase in time of over 5.6 times !!
Why do Turbo-Chargers
make so much Power??.. And Why so much Torque??.. Engines
operate because of cylinder pressure, the amount of energy given off by the explosion of the fuel/air mixture in the combustion
chamber. For example: a cylinder with a bore area on 10 square inches (3.569 inch bore) with 800 psi of pressure
would be subject to a compressive power load of 8000 pound. This means with that 800 psi of pressure, there would
be 8000 lb of force pushing down on the piston, and it's connecting rod. That's a lot of force. With a
Turbo-Charged engine it is very easy to surpass this 800 psi threshold. Turbo-Charged engines can make up to 1600 psi
of cylinder pressure, which is double the stock pressure. Even at 1200 psi, you can see that the same engine with the
same bore area, would now have a compressive load of 12,000 lbs. Thats a huge amount of force pushing down on the piston.
This is why Turbos make so much torque, the extra cylinder pressure directly equates to more compressive power and that to
more torque. While turbos are famous for extra Horse-Power, It's the Torque that really makes them fun.
Understanding
an A/R Ratio - basically the A/R is nothing more then a comparison of exhaust flow,intake volume verses discharge
area. Our A/R ratio is .63
This is
a Compressor Map of our Turbo-Charger, you can plot the map by simply plugging in our figures. As you will see, this
Turbo will work very well for our application. To make any sence out of this chart, we must first
convert or CFM figures to LB/MIN. To do this we multily the CFM by 0.069. or LB/MIN = CFM x 0.069 Engine (a) LB/MIN = 341.38 x 0.069 = 23.55 23.55 / 2 = 11.77 lb/min P/R = 1.49
Engine (b) LB/MIN = 487.04 x 0.069 = 33.60 33.60 / 2 = 16.80lb/min P/R = 1.994
Because we are useing two (2) Turbo-Chargers, we will have to split the CFM rating or you can just split the LB-MIN rating. 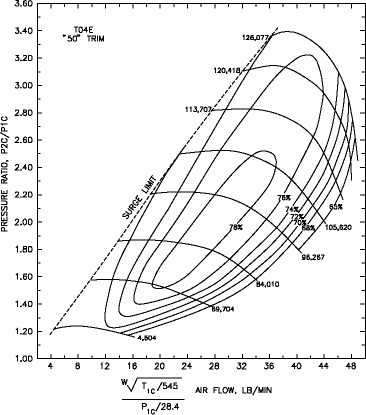
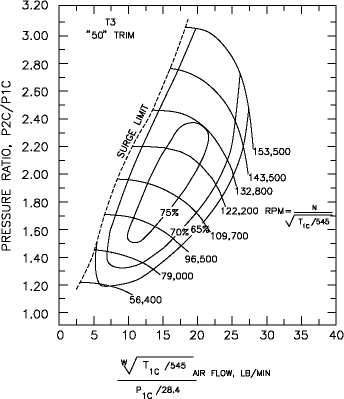
Too plot the Compressor map simply find the right P/R on the left side of the map and the appropiate LB/MIN figure on the
bottom. Extend the two lines and where the contact each other, is the spot at which you'll find their capacity and
the opperating RPM at which they will be. It doesen't take an expert to see that the top map (which is of the compressor
we have) is not exactly ideal for our engine. By contrast, the compressor map of the much smaller T3 compressor is much
closer to a match for our purposes. To correct this, we will change out the compressor housing with a smaller T04E "40".
This will put us back into the area we need to be.
|
 |
This concluded our calculations for Project:Flat-Head, we now have everything needed to install the correct Turbo-Charger and make any necessary
adjustments so the system works at it's best. The following Definitions, Tables, formulas and Information is provided
for your use and or entertainment. Hopefully this will answer the many questions one might have. The following
provided by Mega-Squirt, is from there website and included because they make a wonderful product that allows many people
to convert from a carbureted engine to EFI at a reasonable cost, with all the advantages and adjustability of the more higher
priced systems. Don't follow the crowd and think just because a system is less expensive, that is is in some way
lacking sophistication. Mega squirt, offers everything needed to run, adjust and tune a EFI engine, turbo-charged or
not, and has been proven on road-race circuits, drag strips and city streets all across the USA. FORMULAS, DEFINITIONS and TABLES
Additional information about rigid media:
Virtually all formulas and tables presented here have to do with how the
media performs. It's cooling efficiency, static pressure drop across the media relative to the face velocity and
cubic feet per minute of air flow is the measuring rod to which we apply all other calculations and determinations. In
simpler terms, the rigid media is the heart and soul of evaporative cooling. Without an understanding of it's operation,
it is difficult to design a cooling system or size cooling equipment for a building. The part about applications and design are covered in the section "Applications and Design"
which can be reached from the Technical Data section which is available from our home page. To keep a surprisingly complex
subject simple, only the formulas and tables that relate to this media are covered in this section. Abbreviations
and Definitions: Let's start
with some abbreviations we use in our formulas: AC = Air Changes (
Usually expressed in changes per hour or per minute. Air change is the number of times the air within a structure is
exhausted and replaced during a specified period such as hour or minute). Absolute Pressure
- This term refers to th pressure measured on the scale that
has it's zero point at approximately 14.7 psi (at sea level) below atmospheric pressure. it is a true measurement
of all the pressure, rather than just the pressure above atmospheric. Absolute Temperature -
Similar to absolute pressure, absolute temperature has its zero point where no heat exists. This is approximately 460°f
below 0°f. An absolute degree is the same size as a Fahrenheit degree. The freezing point of water (32°f)
is about 492°f above absolute zero, or 492° absolute. Air/Fuel
Ratio (AFR) - AFR is the ratio of the weight of
air to the weight of fuel in a combustible mixture. AFR is critical in the proper functioning of an engine. Ambient -
Ambient refers to the surrounding atmospheric pressure and temperature. Atmospheric - This word has recently taken
on the connotation of an engine operating without any form of super-charger. My lawn mower has an atmospheric engine. Boost - Boost
is the pressure above atmospheric, measured in the intake manifold. Boost Threshold - Same as Boost Point. This is
the lowest engine RPM at which the turbo-charger will increase power over the engines atmospheric equivalent. More simply
put, the lowest RPM at which noticeable boost can be achieved. Bypass Valve - The bypass valve permits a bleed of flow
around the turbo-charger when the engine is under boost. BTUH = British
Thermal Units per Hour. A measure of heat or the absence of heat ("cold" can be defined as the absence
of heat) in a volume of air or space. BTUH is not commonly used in evaporative cooling terminology but necessary to calculate
heating and mechanical refrigeration. (It is most often used as "heat of vaporization = 1043 BTU/lb in the formula
for calculating evaporation rate and standard CFM). Clearance Volume - Combustion chamber volume above the piston at top dead center is called clearance
volume. (S)CFM = (Standard) Cubic Feet per Minute. Usually referred to as simply CFM. This is a
necessary ingredient in any formula involving evaporative cooling. It is a measure of air volume movement in one minute. Compression Ratio -
This is the displacement volume plus clearance volume divided by the clearance volume. Compressor - For
this writing, the compressor is the air pump itself. The front half of the turbo-charger, through which the intake air
passes. it is also frequently refereed to as the " Cold side" of the turbo-charger. Compressor Efficiency (Ec) - Efficiency is the ratio of what really happens to what should
happen. In the case of the compressor, measurement of the temperature gain caused by compressing the air exceeds what
thermodynamic says it should be. Compressor efficiency converts calculated temperature gains to real temperature gains. Compressor Surge -
Compressor surge occurs when the throttle is slammed shut and air is caught between a pumping turbo and the throttle plate.
This air blasts its way backward out the front of the turbo-charger. When this happens, there is a suddenly room for
more air in the manifold, and air is pumped back in my a still pumping turbo-charger. The throttle is still closed,
so the air again blast back out through the front of the turbo. This continues until the turbo losses enough speed for
leak back around the compressor to dampen the air oscillations. Compressor surge can als occur under boost, if too much
boost pressure is present with low airflow through the system. The chirping sound heard from the turbo when lifting
off the throttle while operating under boost results from the oscillating air volume. This noise is suppressed by the
bypass valve. Crossover point - This is the point at which manifold boost pressure equals turbine inlet pressure. Detonation -
Detonation is spontaneous combustion of the air/fuel mixture ahead of the flame front. When pressure and temperature
exceeds that required for controlled combustion, the mixture auto-ignites. The metallic pinging sound is the resulting
explosion's shock wave colliding with the cylinder walls. Design - This term is used in many ways to define the parameters of an
application or specifications. Some common uses are as follows: IDb = Indoor Dry Bulb. ODb = Outdoor
Dry Bulb. IWb = Indoor Wet Bulb. OWb = Outdoor Wet Bulb. EDb = Entering Dry Bulb. LDb = Leaving Dry
Bulb. EWb = Entering Wet Bulb. LWb = Leaving Wet Bulb. This term is often used in conjunction with "conditions"
such as "Climate Design Conditions". In evaporative cooling, climate data is considered to be Dry Bulb
and Wet Bulb levels. It would require a "Psychometric Chart" to locate the juncture of the Dry Bulb
and Wet Bulb lines to find the grains or pounds of moisture per pound of dry air or relative humidity (RH). Refer to
Table 1 for a psychometric chart digitalized for easy reading of relational elements of Db, Wb and RH. Displacement Volume - is defined in several ways: 1. The swept volume of the cylinder; 2. The
area of the bore times the length of the stroke; 3. Total engine displacement divided by the number of cylinders. Draw-Through -
This indicates that the throttle is on the inlet side of the turbo compressor. Dry Bulb temperature (Db or DB) - Measurement
(usually in Fahrenheit) of temperature taken by a standard thermometer or similar thermal indicator. End Gas - The
end gas is the last part of the air/fuel mixture to burn. It's importance to a turbo-charged engine is paramount,
because it is this end gas in which detonation usually occurs. External Static Pressure - Expressed in inches, water column. The pressure against
which the air flow must move. The pressure external to the cooling unit opposing air flow (i.e. restrictive ductwork,
etc.) Fahrenheit (f or F) - Temperature conforming to a thermometric scale on which water boils at
212 degrees and freezes at 32 degrees. Named after Gabriel D. Fahrenheit, 1736. Fv (or) FV = Face Velocity - Face velocity or "air
velocity" is the measure expressed in feet per minute (FPM) the air is moving at the entry side (face) of the cooling
media. This is another necessary ingredient in any formula in evaporative cooling to determine efficiency. FPM = Feet Per Minute -
The measure of speed (velocity) of the air . Gallons per Hour (GPH) - A measure of liquid (usually water) moving
during one hour. Gallons per Minute (GPM) - A measure of liquid (usually water) moving in one minute. IN. HG - This phrase reads
" Inches of mercury" and is the measure of pressure on yet a different scale. In this project, in-Hg
will reefer to vacuum in the intake manifold, and the scale works downward toward atmospheric pressure. For example,
idle speed vacuum is usually about 18in.Hg, and as throttle is applied, the vacuum goes toward 0 gauge, which is atmospheric
pressure.
Inertia
- The tendency of a body to resist acceleration; the tendency of a body at rest
to remain at rest or of a body in straight line motion to stay in motion in a straight line unless acted on by an outside
force. Resistance or disinclination to motion, action, or change. Inter-cooler -
An inter-cooler is a heat exchanger placed between the turbo and the engine to remove heat from the air exiting the turbo-charger
when operating under boost. Inter-cooler are also called charge air coolers. Inter-cooler Efficiency (EI)
- An Inter-cooler's efficiency is measured by how much heat it removes relative to the heat added
by the compressor. Inertial Load - Internal loads are those created by weight and acceleration. A heavier
piston creates a greater inertial load. likewise, an increase in RPM means greater acceleration and, thus a greater
inertial load. Lag
- Lag is the delay between a change in throttle and the production of noticeable boost when engine RPM
is in a range in which boost can be achieved. Lean - lean means not enough fuel to achieve the correct
air/flue ratio for the existing conditions. Non-sequential
Fuel Injection - EFI that pulses independently of valve position is non sequential. OEM - Original Equipment
manufacturer; the company the built it in the first place. Power
- Strictly speaking, power is the result of how fast a certain amount of work is done. In automotive context,
power is the product of torque at any specific RPM times that RPM. Power Load
- This is the load induced into all engine components by pressure created by the burning gases. Pre-Detonation - This is a meaningless phrase and should
not be included here or anywhere else. Pre-Ignition
- Pre-ignition refers to spontaneous combustion of the air/fuel mixture prior to the spark. Pressure ratio - The ratio of absolute boost
pressure to atmospheric pressure. Pulse Duration -
The amount of time, measured in thousandths of a second (msec), that an electronic fuel injector is held open on any single
pulse. Pulse duration is a relative measurement of the amount of fuel delivered to one cylinder per combustion cycle. Reversion - Reversion occurs when some of the burned
exhaust gases are pushed back into the combustion chamber and intake system during valve overlap. This is caused
by exhaust manifold pressure exceeding intake pressure or by shock waves in the exhaust ports and manifold. Relative Humidity (RH) - Expressed in percent.
The percent of water vapor in the air compared to the amount of water vapor the same air could contain. (i.e.
15% RH indicates the air is 15% saturated with water vapor) Rich
- A condition that exist when too much fuel is present to achieve a maximum -power air/fuel ratio. Saturation Efficiency (SE) - This is the
percent of the Wbd (Wet Bulb Depression) achieved by the cooling process. I.E. At 100 degrees (f) Dry Bulb and 70 degrees
(f) Wet Bulb, the Wbd would be 30 degrees (f). If the actual temperature drop measured at the discharge side of the
media was 73 degrees (f), the percent of saturation efficiency would be 90%. This means that the air passing through
the media has been saturated with water vapor (moisture) to 90% of its maximum. "Cooling Efficiency" is the
same as Saturation Efficiency and is most often used to define the performance level of the media. Also called just
"efficiency". Sequential Fuel Injection -
A fuel injection pulse timed to discharge fuel when the intake valve is in the most advantageous position is called sequenced.
It pulses the injectors in the same sequence as the firing order. Static Pressure Drop - Expressed
in inches, water column. The amount of pressure required to push the air through the media as measured with a magnehelic
gage. The difference between the pressure of the air flow at the intake of the media and the discharge side of
the media. The measure of pressure for any component through which air flow is measured at the intake and discharge. This
is an important consideration in some evaporative cooling applications. Supercharge -
To force more into an engine than the engine can breathe by itself is to supercharged it. A supercharger is a device
that does this, it may be driven by belts, gears or a turbine. When driven by a turbine, it's called turbocharging. Thermal Load - On this site, we will take the rather
narrow definition of heat added to the system by the turbo-charger. This comes from heat produced in the air that is
compressed by the turbo and the mixture heat increase due to reversion. Throttle Response - A change in the speed and torque of an engine brought about by change in
the throttle position is called throttle response. Torque -
The amount of twisting force provided by a turning shaft is called torque. It is measured in foot-pounds, inch-pounds
and even Newton-meters. Turbine - The
turbine is a fan driven by the engine's exhaust gases. it is often called the "hot" side of the turbo-charger. Turbo-Charger - A turbo-charger is a super charger driven
by a turbine. Under Boost - When a system
has greater than atmospheric pressure in the intake manifold, it is operating under boost. Volumetric Efficiency (ev) -
This is the ratio of the number of molecules of air that actually get into a combustion chamber to the number of molecules
in an equal volume at atmospheric pressure. Waste-gate - The waste-gate is a boost-pressure -activated valve that
allows only enough exhaust gas into the turbine to achieve desired boost. The waste-gate routes the remainder of the
exhaust gas around the turbine and out the tailpipe. Water
gauge or Water Column in Inches ( WG or w.g. or WC or w.c.) This is a
measure of static pressure. A Pilot Tube is used to take this measurement. The Pitot Tube is a curved (U-shaped)
glass tube with a prescribed amount of water and a scale. The tube is hollow. When air is blown into one end the water
column will be forced up the other side to some level. The level to which the column of water rises is a measure, in
inches, of the pressure of the force required. Wet Bulb Temperature
(wb or WB) - The lowest temperature that can be reached by evaporatively cooling the air. This
measurement is usually taken with a "sling psychrometer". This device is a standard thermometer with a "wet
sock" over the sensor bulb. The psychrometer is slung in a circular motion rapidly enough to cause evaporation
to occur around the sensor bulb to drop the temperature to it's lowest point possible with the evaporation process. Wet Bulb Depression (wbd or WBD) - The difference between the Dry Bulb and the Wet Bulb
temperatures. This temperature is the total amount of cooling available through the evaporative cooling process. At
100% cooling efficiency, the temperature drop would be equal to the Wet Bulb Depression. Also known as Wet Bulb Differential.
|
 |
Leaving Dry Bulb = [ODb - (SE x (Odb-OWb)] Leaving Wet Bulb =
Normally considered same as entering Wb. Wet Bulb Depression = ODb - OWb Evaporation Rate =
[CFM x WBd x (SE / 8700)] ( this is simple method) Bleed - Off Rate = Evaporation
Rate x .20 (prox) (Recirculation) Water flow Rate = 3 times the evaporation rate (prox) Standard
CFM = Sensible BTU/hr / (1.08 x (IDb - Db) x Density Ratio Where
IDb = Indoor Design Dry Bulb (f) CFM = Standard CFM / Density Ratio BTU = CFM
X Delta T x 1.08 Density Ratio = 1.325 x Barometric Pressure / (Db(f)
Water weight (US gallon) = 8.33 pounds per gallon (based on distilled water) Water
volume (US gallon) = 7.481 gallons per cubic foot Water weight (US gallon cubic foot) =
7.481 x 8.33 = weight of cubic foot of water (62.288#) Face Area = Width x Height
of open face area through which air will flow (expressed in square feet.) Face
Velocity = CFM / Face Area (Sq Ft) (expressed in Feet per minute (FPM).
Table 1: Psychrometric Chart #H20 per #Dry Air | Temperature (Dry Bulb degrees f) |
---|
40 | 50 | 60
| 70 | 80 | 90 | 100 | 110 | 120 |
---|
Wb | RH% | Wb | RH% | Wb | RH% | Wb | RH% | Wb | RH% | Wb | RH% | Wb | RH% | Wb | RH% | Wb | RH% |
---|
.001 | 27 | 20 | 34
| 12 | 41 | 10 | 46 | 8
| 51 | 5 | 54 | 3 | 57
| 2 | 62 | 2 | 65 | 1
| .002 | 32 | 40 | 36 | 28
| 43 | 19 | 47 | 12 | 53
| 10 | 57 | 7 | 60 | 5
| 63 | 4 | 66 | 2 | .003
| 35 | 58 | 41 | 40 | 45
| 28 | 50 | 19 | 54 | 15
| 58 | 10 | 62 | 7 | 65
| 6 | 67 | 3 | .004 | 36 | 75
| 43 | 51 | 47 | 38 | 52
| 26 | 56 | 19 | 59 | 13
| 63 | 10 | 66 | 8 | 69
| 5 | .005 | 39 | 95 | 45
| 65 | 50 | 47 | 54 | 32
| 57 | 23 | 61 | 17 | 64
| 12 | 67 | 9 | 71 | 7
| .006 | x | x
| 46 | 78 | 51 | 55 | 55
| 39 | 59 | 27 | 63 | 20
| 66 | 15 | 69 | 10 | 72
| 9 | .007 | x | x | 49 | 91 | 53 | 64
| 57 | 45 | 61 | 31 | 65
| 24 | 67 | 18 | 70 | 12
| 73 | 10 | .008 | x
| x | x | x | 55 | 73 | 59 | 51
| 63 | 36 | 66 | 28 | 68
| 20 | 71 | 14 | 74 | 11
| .009 | x | x
| x | x | 56
| 82 | 60 | 57 | 64 | 41
| 67 | 30 | 70 | 22 | 72
| 16 | 75 | 13 | .010 | x
| x | x | x | 57 | 90 | 62 | 63
| 65 | 46 | 68 | 33 | 72
| 25 | 74 | 18 | 76 | 14
| .011 | x | x
| x | x | 60
| 99 | 64 | 70 | 66 | 50
| 70 | 36 | 73 | 27 | 76
| 20 | 77 | 15 | .012 | x
| x | x | x | x | x
| 65 | 76 | 67 | 55 | 71
| 40 | 74 | 29 | 77 | 22
| 78 | 17 | .013 | x
| x | x | x | x | x
| 66 | 83 | 69 | 59 | 72
| 44 | 75 | 31 | 78 | 24
| 80 | 19 | .014 | x
| x | x | x | x | x
| 67 | 90 | 70 | 63 | 74
| 47 | 76 | 33 | 78 | 26
| 81 | 20 | .015 | x
| x | x | x | x | x
| 69 | 95 | 72 | 68 | 75
| 50 | 77 | 36 | 79 | 27
| 82 | 21 | .016 | x
| x | x | x | x | x
| 70 | 99 | 73 | 72 | 76
| 53 | 78 | 39 | 81 | 29
| 83 | 22 | .017 | x
| x | x | x | x | x
| x | x | 74
| 76 | 77 | 57 | 79 | 42
| 82 | 31 | 83 | 23 | .018
| x | x | x | x | x
| x | x | x | 75 | 80 | 77 | 59
| 80 | 45 | 82 | 33 | 84
| 24 | .019 | x | x | x | x
| x | x | x | x | 76 | 85
| 78 | 62 | 81 | 47 | 83
| 35 | 85 | 25 | .020 | x
| x | x | x | x | x
| x | x | 77
| 90 | 80 | 65 | 82 | 49
| 84 | 37 | 86 | 27 | .021
| x | x | x | x | x
| x | x | x | 78 | 95 | 81 | 69
| 83 | 51 | 85 | 39 | 87
| 28 | .022 | x | x | x | x
| x | x | x | x | 79 | 99
| 82 | 72 | 84 | 53 | 86
| 40 | 87 | 29 | .023 | x
| x | x | x | x | x
| x | x | x | x | 83 | 75
| 85 | 55 | 87 | 41 | 88
| 31 | .024 | x | x | x | x
| x | x | x | x | x
| x | 84 | 78 | 86
| 58 | 88 | 42 | 90 | 33
| .025 | x | x
| x | x | x | x | x
| x | x | x | 85 | 81 | 87 | 60
| 89 | 43 | 91 | 34 | .026
| x | x | x | x | x
| x | x | x | x | x
| 86 | 85 | 88 | 62 | 90
| 44 | 92 | 35 | .027 | x
| x | x | x | x | x
| x | x | x | x | 87 | 88
| 89 | 65 | 91 | 46 | 93
| 36 | .028 | x | x | x | x
| x | x | x | x | x
| x | 88 | 91 | 90
| 67 | 92 | 47 | 94 | 37
| .029 | x | x
| x | x | x | x | x
| x | x | x | 89 | 95 | 91 | 69
| 93 | 49 | 95 | 39 | .030
| x | x | x | x | x
| x | x | x | x | x
| 90 | 99 | 92 | 71 | 94
| 51 | 95 | 40 |
Notes: Db is Dry Bulb temperature, Wb is Wet Bulb temperature, #H2o is Pounds
of moisture per pound of dry air which is a measure of absolute humidity. RH is Relative Humidity. Read the chart by finding the known elements, such as Dry Bulb and Relative
Humidity and move horizontal to find the Wet Bulb and pounds of moisture per pound of dry air. I.E. The weather forecast
is for 100 degrees at 15% Relative Humidity. You will find that the Web Bulb is 66 degrees and the pounds of moisture
is .006 per pound of dry air. Knowing the Wet Bulb will allow you to determine the Wet Bulb depression. The Psychometric chart will provide you the necessary information to design
systems, predict outcomes and many other useful applications of the information! This chart is digitalized to make it
simpler to use. It is necessary to interpolate
and extrapolate accordingly for those in-between conditions not directly covered in the above chart. I.E. if 105 degrees
was the temperature to use, then it would be necessary to interpolate the available data to reach the right conclusion. In
this instance, the Wet Bulb would be 67.5 degrees. This chart is intended to be reasonably accurate at sea level and
should be within p/m 5%. If greater accuracy is required, it is recommended that you use the proper Psychometric chart
for the elevation desired or make proper adjustments for elevation (barometric pressure). Table 2: Evaporation
Rate
Wbd(f)
| Gallons Per Hour evaporated per 1000
CFM with a Saturation Efficiency of: |
---|
0.80 | 0.82 | 0.84 | 0.86 | 0.88 | 0.90
| .092 | 0.94 | 0.96 | 0.98 |
---|
5 | 0.50
| 0.51 | 0.52 | 0.53 | 0.55 | 0.56 | 0.57 | 0.58
| 0.60 | 0.61 | 10 | 0.99 | 1.02
| 1.04 | 1.07 | 1.09 | 1.12 | 1.14 | 1.17 | 1.19
| 1.22 | 15 | 1.49 | 1.53 | 1.56
| 1.60 | 1.64 | 1.68 | 1.71 | 1.75 | 1.79 | 1.83
| 20 | 1.99 | 2.04
| 2.09 | 2.14 | 2.19 | 2.23 | 2.28 | 2.33 | 2.38
| 2.43 | 25 | 2.48 | 2.55 | 2.61
| 2.67 | 2.73 | 2.79 | 2.86 | 2.92 | 2.98 | 3.04
| 30 | 2.98 | 3.05
| 3.13 | 3.20 | 3.28 | 3.35 | 3.43 | 3.50 | 3.58
| 3.65 | 35 | 3.48 | 3.56 | 3.65
| 3.74 | 3.82 | 3.91 | 4.00 | 4.08 | 4.17 | 4.26
| 40 | 3.97 | 4.07
| 4.17 | 4.27 | 4.37 | 4.47 | 4.57 | 4.67 | 4.77
| 4.87 | 45 | 4.47 | 4.58 | 4.69
| 4.80 | 4.92 | 5.03 | 5.14 | 5.25 | 5.36 | 5.48
|
To determine Gallons
per Minute divide by 60. Formula to determine evaporation rate is shown in Formulas section. Table 3: Temperature drop across media (@ 500 FPM face velocity)
Wbd (f) | Temperature drop (Dry Bulb) for Media Thickness of: |
---|
4" | 6" | 8" | 12" | 18" | 24"
|
---|
10.0 | 5.3 | 6.8
| 7.9 | 8.9 | 9.8 | 9.9
| 12.5 | 6.6 | 8.5
| 9.8 | 11.1 | 12.2 | 12.3 | 15.0
| 7.9 | 10.2 | 11.8
| 13.3 | 14.6 | 14.8 | 17.5 | 9.2 | 11.9 | 13.8
| 15.6 | 17.1 | 17.3 | 20.0 | 10.5 | 13.6 | 15.8
| 17.8 | 19.5 | 19.7 | 22.5 | 11.8 | 15.3 | 17.7
| 20.0 | 21.9 | 22.2 | 25.0 | 13.2 | 17.0 | 19.7
| 22.2 | 24.4 | 24.7 | 27.5 | 14.5 | 18.7 | 21.7
| 24.4 | 26.8 | 27.2 | 30.0 | 15.8 | 20.4 | 23.6
| 26.7 | 29.3 | 29.6 | 32.5 | 17.1 | 22.1 | 25.6
| 28.9 | 31.7 | 32.1 | 35.0 | 18.4 | 23.8 | 27.6
| 31.1 | 34.1 | 34.6 | 37.5 | 19.7 | 25.5 | 29.5
| 33.3 | 36.6 | 37.0 | 40.0 | 21.1 | 27.2 | 31.5
| 35.6 | 39.0 | 39.5 |
Note: 12" thick media @ 500 FPM face velocity is the
preferred design . This is the best trade-off between performance and cost. Table 3: Air Density Ratio:
Density Ratio for Various Elevations and Temperatures.
Temp. | Elevation/Inches Hg |
---|
(f) | 0/ 29.92 | 1000/ 28.86 | 2000/ 27.82 | 3000/ 26.82 | 4000/ 25.84 | 5000/ 24.90 | 6000/ 23.98 | 7000/ 23.09 | 8000/ 22.22 | 9000/ 21.39 | 10000/ 20.58 |
---|
68
| 1.00 | 0.97
| 0.93 | 0.90
| 0.87 | 0.84
| 0.80 | 0.77
| 0.75 | 0.72
| 0.69 | 70
| 1.00 | 0.96
| 0.93 | 0.90
| 0.86 | 0.83
| 0.80 | 0.77
| 0.74 | 0.71
| 0.69 | 72
| 1.00 | 0.96
| 0.93 | 0.89
| 0.86 | 0.83
| 0.80 | 0.77
| 0.74 | 0.71
| 0.69 | 74
| 0.99 | 0.96
| 0.92 | 0.89
| 0.86 | 0.83
| 0.80 | 0.77
| 0.74 | 0.71
| 0.68 | 76
| 0.99 | 0.95
| 0.92 | 0.89
| 0.85 | 0.82
| 0.79 | 0.76
| 0.73 | 0.71
| 0.68 | 78
| 0.99 | 0.95
| 0.92 | 0.88
| 0.85 | 0.82
| 0.79 | 0.76
| 0.73 | 0.70
| 0.68 | 80
| 0.98 | 0.95
| 0.91 | 0.88
| 0.85 | 0.82
| 0.79 | 0.76
| 0.73 | 0.70
| 0.68 |
Table
4: Air Changes suggested per hour.
Leaving Air Temp (LDb) |
| Temperature
over outside ambient |
| Air
Changes Per Hour |
---|
| | Over 78 (f) | | 20+ | | 30-60
| 76f to 78f | | 15 to 20 | | 20 to 40 | 74f
to 76f | | 10
to 15 | | 15
to 30 | 72f to 74f | | 5 to 15 | | 12 to 20 | Less than 72f
| | Less than 10
| | 10 to 15
|
Notes: The
"Air Change" method is a practical approach to assist in the determination of the size and efficiency of evaporative
cooling equipment required for the structure. The principle behind this method is to determine the difference between
the inside temperature of the structure, without using evaporative cooling and the outside ambient temperature during its
highest condition. While this method is ideal for existing structures, new structures not yet built can be estimated
on the same scale. Leaving Air Temperature reflects
the output of the evaporative coolers whether existing or planned. The air change column indicates a range of frequency
and is used in determining air volume requirements. Other criteria are needed to complete the sizing of equipment. Refer
to the section on "Applications and Design" for more specific information on equipment sizing. Acronyms / Terminology A/R
A/R
describes a geometric characteristic of all compressor and turbine housings. It is defined as the inlet cross-sectional area
divided by the radius from the turbo centerline to the centroid of that area. Compressor A/R - Compressor performance is largely insensitive
to changes in A/R, but generally larger A/R housings are used to optimize the performance for low boost applications, and
smaller housings are used for high boost applications. Usually there are not A/R options available for compressor housings.
Turbine
A/R - Turbine performance is greatly affected by changing the A/R of the housing. Turbine A/R is used to adjust the flow capacity
of the turbine. Using a smaller A/R will increase the exhaust gas velocity into the turbine wheel, causing the wheel to spin
faster at lower engine RPMs giving a quicker boost rise. This will also tend to increase exhaust back pressure and reduce
the max power at high RPM. Conversely, using a larger A/R will lower exhaust gas velocity, and delay boost rise, but the lower
back pressure will give better high RPM power. When deciding between A/R options, be realistic with the intended vehicle use
and use the A/R to bias the performance toward the desired power-band.
Choke Line The choke
line is on the right hand side of a compressor map and represents the flow limit. Properly sizing a turbo is important to
prevent the compressor from operating past the choke line. When a turbocharger is run deep into choke, turbo speeds increase
dramatically while compressor efficiency plunges (very high compressor outlet temps). Additionally, the turbo's durability
is compromised by the resulting high thrust loads.
CHRA
(Center Housing & Rotating Assembly) The CHRA is essentially a turbocharger minus the compressor
and turbine housings
Clipped Turbine Wheel When an angle is machined on the turbine wheel exducer (outlet side), the wheel is said to be "clipped".
Clipping causes a minor increase in the wheel's flow capability; however, it dramatically lowers the turbo efficiency.
This reduction in efficiency causes the turbo to come up on boost at a later engine speed (ex. increased turbo lag). High
performance applications should never use a clipped turbine wheel. All Garrett GT turbos use modern unclipped turbine wheels.
Corrected
Air Flow When plotting actual airflow
data on a compressor map, the flow must be corrected to account for different atmospheric conditions that affect air density.
Example: Air Temperature (Air Temp) - 60°F Barometric Pressure (Baro) - 14.7 psi Engine air consumption (Actual Flow) = 50 lb/min Corrected Flow= Actual Flow SQR([Air Temp+460]/545)/ Baro/13.95 Corrected Flow= 50*SQR([60+460]/545)/ 14.7/13.95 = 46.3 lb/min
Efficiency Contours The efficiency contours
depict the regional efficiency of the compressor stage. When sizing a turbo, it is important to maintain the proposed lug-line
with a high efficiency range on the map. Free-Float A free-floating turbocharger has no Waste-gates device. This turbocharger can't control its own boost
levels. For performance applications, the user normally must install an external Waste-gates.
GT
The GT designation
refers to Garrett's state-of-the-art turbocharger line. GT-series turbos use redesigned bearing systems and modern compressor/turbine
aerodynamics. These new compressor and turbine wheels represent huge efficiency improvements over the old T2, T3, T3/T4, T04
products. The net result is increased durability, higher boost, and more engine power over the older T-series product line.
On-Center
Turbine Housings On-center turbine housings refer to an outdated style of turbine housing with a centered turbine inlet pad.
The inlet pad is centered on the turbo's axis of rotation instead of being tangentially located. Using an on-center housing
will significantly lower the turbine's efficiency. This results in increased turbo lag, more back-pressure, lower engine
volumetric efficiency, and less overall engine power. No Garrett OEM's use on-center housings.
Pressure Ratio Ratio
of absolute outlet pressure divided by absolute inlet pressure Example: Intake manifold pressure (Boost)
= 12 psi Pressure drop, inter-cooler (DPIntercooler) = 2 psi Pressure drop, air filter (DPAir Filter)
= 0.5 psi Atmosphere (Atmos) = 14.7 psi at sea level PR = (Boost + DPIntercooler+ Atmos) / (Atmos-DPAir
Filter) PR = (12 + 2 + 14.7) / (14.7 -.5) = 2.02
Surge Line The surge region, located on the left-hand side of the compressor map, is an area of flow instability typically
caused by compressor inducer stall. The turbo should be sized so that the engine does not operate in the surge range. When
turbochargers operate in surge for long periods of time, bearing failures may occur.
Trim
Trim
is an area ratio used to describe both turbine and compressor wheels. Trim is calculated using the inducer and exducer diameters.
Example: Inducer diameter = 88mm Exducer diameter = 117.5mm Trim = Inducer2/Exducer2 Trim =
882/117.52 = 56 Trim As trim is increased, the wheel can support more air/gas flow.
Waste-gates A Waste-gated turbocharger includes an integral
device to limit turbo boost. This consists of a pneumatic actuator connected to a valve assembly mounted inside the turbine
housing. By connecting the pneumatic actuator to boost pressure, the turbo is able to limit its maximum boost output. The
net result is increased durability, quicker time to boost, and adjustability of boost.
Injectors
and Fuel System In
order to make your Mega Squirt work on a vehicle, you will need the following additional fuel system items to suit your installation: - injectors and bungs/manifold,
- throttle body,
- high pressure fuel pump, supply/return lines, and rails,
- and a fuel pressure
regulator.
It provides each injector bank with a separate
|
|
Injector-Size = (Horse Power
* BSFC) / (#Injectors * Injector-Size = (Horse Power * BSFC) / (#Injectors * In order to properly install your Mega Squirt,
you need to select and install fuel system components appropriate for your engine. Most important is that you have fuel injectors
that are the correct size in terms of flow rating. In fact, most injectors are a similar size physically, though there tends
to be more variation in throttle body injection injectors than in port injectors. Typical dimensions for a port injector are:
Injector-Size = (Horse Power
* BSFC) / (#Injectors * In order to properly install your Mega Squirt,
you need to select and install fuel system components appropriate for your engine. Most important is that you have fuel injectors
that are the correct size in terms of flow rating. In fact, most injectors are a similar size physically, though there tends
to be more variation in throttle body injection injectors than in port injectors. Typical dimensions for a port injector are:
Note that if you start by installing
Mega Squirt with a throttle body injection unit from a late model vehicle, it will likely come with the injectors, pressure
regulator, and throttle position sensor; this will greatly simplify the installation of Mega Squirt on a vehicle that was
previously carbureted. If you choose a TBI unit, you will not need as much wiring, fuel rails, manifold modifications for
injector bungs, etc. Once you get the TBI set-up working, you can later switch to port injection and use the TBI as an air
door only.
Injector Selection In order to properly install your Mega Squirt, you
need to select and install fuel system components appropriate for your engine. Most important is that you have fuel injectors
that are the correct size in terms of flow rating. In fact, most injectors are a similar size physically, though there tends
to be more variation in throttle body injection injectors than in port injectors. Typical dimensions for a port injector are:
Injector Selection In order to properly install your Mega Squirt, you
need to select and install fuel system components appropriate for your engine. Most important is that you have fuel injectors
that are the correct size in terms of flow rating. In fact, most injectors are a similar size physically, though there tends
to be more variation in throttle body injection injectors than in port injectors. Typical dimensions for a port injector are:
Injector Selection In order to properly install your Mega Squirt, you
need to select and install fuel system components appropriate for your engine. Most important is that you have fuel injectors
that are the correct size in terms of flow rating. In fact, most injectors are a similar size physically, though there tends
to be more variation in throttle body injection injectors than in port injectors. Typical dimensions for a port injector are:
Injector Selection In order to properly install your Mega Squirt, you
need to select and install fuel system components appropriate for your engine. Most important is that you have fuel injectors
that are the correct size in terms of flow rating. In fact, most injectors are a similar size physically, though there tends
to be more variation in throttle body injection injectors than in port injectors. Typical dimensions for a port injector are:
Injector Selection In order to properly install your Mega Squirt, you
need to select and install fuel system components appropriate for your engine. Most important is that you have fuel injectors
that are the correct size in terms of flow rating. In fact, most injectors are a similar size physically, though there tends
to be more variation in throttle body injection injectors than in port injectors. Typical dimensions for a port injector are:
Injector Selection In order to properly install your Mega Squirt, you
need to select and install fuel system components appropriate for your engine. Most important is that you have fuel injectors
that are the correct size in terms of flow rating. In fact, most injectors are a similar size physically, though there tends
to be more variation in throttle body injection injectors than in port injectors. Typical dimensions for a port injector are:
Injector Selection In order to properly install your Mega Squirt, you
need to select and install fuel system components appropriate for your engine. Most important is that you have fuel injectors
that are the correct size in terms of flow rating. In fact, most injectors are a similar size physically, though there tends
to be more variation in throttle body injection injectors than in port injectors. Typical dimensions for a port injector are:
Injector Selection In order to properly install your Mega Squirt, you
need to select and install fuel system components appropriate for your engine. Most important is that you have fuel injectors
that are the correct size in terms of flow rating. In fact, most injectors are a similar size physically, though there tends
to be more variation in throttle body injection injectors than in port injectors. Typical dimensions for a port injector are:
The
manifold injector bungs are 0.530"-0.535" inside diameter [about 17/32" or 13.5 mm]. The fuel supply rail bungs
for the top of the injectors are the same size. Here
are two TBI injectors. One the left is a Holley 85 lb/hr TBI injectors (apparently similar to some Chrysler TBI injectors),
on the right is a GM TBI injector from a 1984 Corvette: Injectors that have
too large a flow rating will make it difficult to tune the engine at idle and cruise. Injectors that have too small a flow
rating can starve the engine of fuel at full power, and seriously damage your engine. To determine how big your injector's
flow rating should be, multiply estimated horsepower (HP) of your engine by the brake specific fuel consumption (BSFC)* and
divide by the number of injectors and the desired duty cycle and you will get a rough estimate of injector size: Injector-Size = (Horse Power * BSFC)
/ (#Injectors * Duty Cycle) for
example, a 135 horsepower gasoline fueled 4 cylinder engine with 2 throttle body injectors and 0.55 brake specific fuel consumption
gives:
135 HP * 0.55
lb/hr/HP) / (2*.85) = ~ 43.7 lb/hr Injectors rated
between 42 and 45 lb/hr would be okay in this case. *BSFC
is the amount of fuel your engine uses to make 1 horsepower for one hour. It is usually between 0.42 and 0.58 at wide open
throttle. Normally aspirated engines with efficient combustion processes are at the lower end of the BSFC scale [~0.45], supercharged
engines tend to be towards the higher end [~0.55].
Or you can use the following
chart to select injectors based on the total horsepower of your engine and the total number of injectors: Injectors Rating Required
for Specified Horsepower in lbs/hr and (cc/min) |
| Number of Injectors | Horsepower Horsepower | 1 | 2 | 4 | 5 | 6 | 8 | 100 | 59 (620) | 29 (305) | 15
(158) | 12 (126) | 10 (105) | - | 150 | 88 (924) | 44 (462) | 22
(231) | 18 (189) | 15 (158) | 11
(116) | 200 | - | 59
(620) | 29 (305) | 24 (252) | 20
(210) | 15 (158) | 250 | - | 74
(777) | 37 (389) | 29 (305) | 25
(263) | 18 (189) | 300 | - | 88
(924) | 44 (462) | 35 (368) | 29
(305) | 22 (231) | 350 | - | - | 51
(534) | 41 (431) | 34 (357) | 26
(273) | 400 | - | - | 59
(620) | 47 (494) | 39 (410) | 29
(305) | 450 | - | - | 66
(693) | 53 (557) | 44 (462) | 33
(347) | 500 | - | - | 74
(777) | 59 (620) | 49 (515) | 37
(389) | 550 | - | - | 81
(851) | 65 (683) | 54 (567) | 40
(420) | 600 | - | - | 88
(924) | 71 (746) | 59 (620) | 44
(462) | based on 0.50 BSFC and 85% duty cycle Turbo/supercharged
engines should add 10% to listed minimum injector size
|
Injectors are usually rated in either lbs/hour cc/min. The accepted
conversion factor between these depends somewhat on fuel density, which changes with formulation (i.e., by season), but the
generally used conversion for gasoline is: 1 lb/hr ~ 10.5 cc/min
Another way to select injectors is to take
them from an engine that makes nearly the same power as your engine will [assuming the same number of injectors]. If your regulator is adjustable (many aftermarket
ones are), you can also adjust the fuel pressure to achieve different flow rates. The formula is:
new flow rate = old flow rate × SQRT[new
pressure÷old pressure]) So
for example, if you had 30 lb/hr injectors rated at 43.5 psi, and you went to 50 psi, you would get: flow rate = 30 * SQRT(50/43.5)
= 32 lb/hr Do not run more than 70 psi fuel pressure,
or the injectors may not open/close properly. However, do not install injectors with a much larger flow capacity than you need. Very large injectors will
create idle pulse width issues that will make tuning very difficult. You can estimate your idle
pulse width beforehand. For proper tuning, you will need an idle pulse width of at least 1.7 milliseconds. To calculate the
idle pulse width, recall that the fueling equation for Mega Squirt is: PW = REQ_FUEL * VE * MAP * E + accel + Injector_open_time
So, find the REQ_FUEL that corresponds to your
injector's flow rate and engine size. There is a REQ_FUEL calculator in Mega Tune, and also here. If you have the engine running , you can check the MAP at
idle (or you can guess - pick about ~25 kPa for a stock cam, ~35 kPa for a performance cam, ~45 kPa for a race cam). Then
you only need the idle VE (and injector open time) to predict the idle pulse width, since this is minimum when there are no
enrichments (E=0, accel=0). Note that you need to use the 'downloaded' REQ_FUEL, which is adjusted for the number
of injectors and their staging. A
good "rule of thumb" for idle VE is 30%. You may actually be 20% or 40% depending on things like compression, overlap,
ignition timing, etc., but 30% will be close enough to give you a good idea about idle pulse width. And use 1.0 msec for the
injector opening time, unless you have a very good reason to do otherwise. For example, on one engine: PW = 6.3 msec * 30% * (33 kPa / 100 kPa) + 1.0 msec = 1.62
msec And the measured idle PW
was 1.7 msec. So these injectors are okay on this engine, but just barely. If it had been 1.2 or 1.3 milliseconds, these injectors
would have presented very significant tuning problems on this engine. Injectors frequently have identifying numbers stamped on them. You may
be able to identify your injectors by looking on: http://www.geocities.com/MotorCity/Pit/9975/dataBySubject/Injectors.htmlor http://www.telusplanet.net/%7Echichm/tech/injectors.pdf Injectors should not be used at more than
80-85% duty cycle. However, injector rates are always specified at 100% duty cycle and some nominal pressure (usually 43.5
psi = 3 atmospheres). The manufacturer leaves it up to you to determine a system pressure and maximum duty cycle in order
to compute the resulting flow.
Injectors
are driven by an electrical signal from Mega Squirt that grounds the +12 volt supply through the injectors to open them. Once
they are open, they flow at a constant rate until closing. The amount of time required to open and close the injectors is
specified in Mega Squirt as the 'Injector Opening Time' (usually about 1.0 msec). Here is an example of a low impedance
injector's pulse voltage, current, and fuel flow: Pulse Width Modulation Injectors
are either high impedance or low impedance. High impedance injectors (usually about 12-16 ohms) can take a 12 supply directly,
without a form of current control. Low impedance injectors (generally below 3 ohms) require some form of current limiting.
With Mega Squirt, you can use resistors to limit current, or you can use Pulse Width Modulation (PWM), which is a software
solution built in to Mega Squirt. PWM works by switching
the 12 volt ground to the injector on and off very rapidly (in about 0.000059 seconds!). The ratio of the "on" time
to the "off" time determines the current through the injectors. However, the easiest way to think of the PWM% is
as a percentage of the supply voltage, so 50% PWM on a 14 volt supply becomes effectively 7 volts on average, 28% would be
4 volts, etc. Remember
that pulse width and PWM% are two different things. Pulse width is the total duration of the signal whereas PWM% is the ratio
of 'on-time' to 'off-time' within the pulse. So in the above illustration, the pulse width for both is the
same, but the PWM% for the first is 50%, while for the second it is 25%. The PWM% you will be able to use depends on the fly back circuit you have.
Version 2.2 hardware generally requires about 55% to 75% PWM. Often the engine will run with lower values, but will not have
enough voltage to re-start. Note that using embedded code version 2.986 or higher will disable PWM during cranking, allowing somewhat lower PWM% values. The Fly Back Board allows you to lower the PWM% dramatically, generally to 30%
or less. It also helps close the injectors faster. With better fly back control, you can reduce injector opening times (recall that the injector
opening time is really the sum of the opening and closing times), and increase the duration of the 'controllable'
part of the pulse width (i.e. after the opening time), The important thing about the injector open time is that it sets a lower bound for the pulse
width (regardless of whether PWM is on, etc.). so if you have injector opening at 1.7ms, you cannot set it to 1.6 or anything
lower, even with VE=0. Mega Squirt assumes no fuel is injected during this time, but some is, though it is hard to calculate
how much. The longer it takes to open, the more fuel is likely injected during opening. With lower opening times (by allowing
full voltage (i.e. no PWM), you can get the injectors open quicker. Your engine will need a certain amount of fuel to run correctly at idle when fully warmed
up. If this amount is below that injected during the injector opening time, you will always be rich and have no way to lean
it out, short of reducing the fuel pressure. Note
that PWM is disabled (in v2.986 code) during cranking so the injectors get full battery voltage. This makes 'severe'
starting conditions (lower cranking voltages, etc.) less likely to result in the injectors not opening. This is not possible
with resistors, unless you devise a way to bypass them during starting (like the older cars did for the ignition coil).
When using low-impedance injectors, which are also called peak and hold injectors (P&H), you wire them in parallel. The
wiring is the same for P&H or saturated [high-impedance]. To
exceed the recommended number of injectors (see below) either requires resistors in series with each injector or
a modified fly back setup. The following is a guide as to whether you need to use resistors or the fly back board: To exceed the recommended number of injectors (see below) either requires resistors in series with
each injector or a modified fly back setup. The following is a guide as to whether you need to use resistors or the fly back board: To exceed the recommended number of injectors (see below) either requires resistors in series with
each injector or a modified fly back setup. The following is a guide as to whether you need to use resistors or the fly back board:
Injector DC Resistance | Number of Injectors (total) | Mega Squirt Hardware | PWM Mode | High
(12 - 16 ohm) | up to 12 | V2.2 | no PWM current limit | Low
(> 2.4 ohm) | up to 4 | V2.2 | use PWM current limit | Low
(> 2.4 ohm) | more than 4 | V2.2 | Use injector resistors or fly back board | Low (< 2.4 and > 1.2 ohm) | more than 3 | V2.2 | Use injector resistors or fly back board | Low (< 1.2 ohm) | up to 2 | V2.2 | use
PWM current limit | Low (< 1.2 ohm) | more than 2 | V2.2 | Use injector resistors or fly back board |
One sure way to know if you can't use the standard V2.2
fly back circuit is to have a fly back failure. The circuit will most often fail after some time spend at high speeds and
loads, rather than immediately when you start the engine for the first time. Generally, when the fly back circuit fails, the
Mega Squirt works okay on the stim, but not on the car. - Mega Squirt will often require higher PWM%
over time,
- The engine may
start running erratically, especially at higher speeds and loads,
- the injectors may 'stick' open and flood the engine.
Signs of an impending fly back failure are: Signs of an impending fly back failure are:
Pro Weld
5937 Ethan Drive
Burlington, KY 41005
859-586-4069
Proweld@myway.com
|
|
|
 |